Washington, DC - In an essay titled “The end of artefacts, ” Nobel laureate and National Institute of Standards and Technology (NIST) fellow William D. Phillips details how scientists came to realize the original vision of the metric system, or the International System of Units (SI) — a system of units “for all times, for all people.” With the redefinition of the kilogram in 2019, the new SI was rightly celebrated as a unifying achievement toward the democratization of science, with NIST and its international partners having collectively led the charge.
But while the polished platinum and iridium cylinder nicknamed Le Grand K (The Big K) no longer defines the base unit of mass, it and many other physical artifacts still play a vital role in establishing the continuity of measurement chains, an arrangement called traceability, in important areas of commerce and trade. For example, artifacts are routinely used to study the different varieties of atoms, or isotopes, that make up matter. Small differences in isotopic composition can reveal changes in climate and atmosphere, ecology, geochemistry and food networks. In the forensics laboratory, measurements of isotopic composition can give away the illicit trade of materials like ivory or be used to track the origin and movements of unknown murder victims and other evidence.
In all these fields, researchers must be able to make consistently accurate measurements that can be compared across different studies. To do this, they use physical artifacts to calibrate their precision scientific instruments. Inevitably, however, the artifacts required for calibration can experience drifts in their isotopic composition — or get used up entirely during repeated testing. This potential calamity caused my colleague Joseph T. Hodges, a mechanical engineer at NIST, to ask the question: Can artifact-free measurements of isotopic composition be made traceable to the new SI?
A Brief History of an Artifact
Around 1950, during a fossil-hunting expedition to the southeastern United States, Heinz A. Lowenstam and Harold C. Urey collected samples of a marine deposit. That specimen, mined from the banks of the Pee Dee River in South Carolina, became the anchor point for measuring stable isotope ratios of both the elements carbon (C) and oxygen (O). With a reference material like the Pee Dee Belemnite (PDB) sample from Lowenstam and Urey on hand, researchers could measure the isotope ratios of unknown materials relative to the isotope ratio of the PDB specimen. This ratio-of-ratios approach ensured that measurements performed at different laboratories could be reliably compared on what became known as the isotope ratio delta scales, δ 13C and δ 18O.
Seventy years later, the original sample of PDB has long since disappeared, and generations of other reference materials have followed in its wake. At the time of their expedition, Urey had already won the 1934 Nobel Prize in chemistry for detecting a heavy isotope of hydrogen, known as deuterium, with help from Ferdinand Brickwedde of the National Bureau of Standards (NBS, now NIST). In an oral history, Lowenstam describes being on that field trip with his Nobel laureate sidekick, Urey: I had to restrain him at the beginning, because otherwise I couldn’t get much done. He just was all over the place. When we came to the Pee Dee River in South Carolina, where we saw an outcrop of some extinct cuttlefish concentrations, he was ready to grab. I said, “No, Harold. We first take a picture. We number and label each specimen. Because how else do we tell how that deposit’s been laid down over several million years?”
Isotopic Composition Reveals Moments in Time
As Lowenstam alludes to in the above passage, it was important that he and his colleagues understood the geology of the samples that they were collecting from across the eastern United States and Europe. That is because Urey and his team realized that measurements of isotopic composition could reveal something very interesting about specific moments in time. In their case, the isotopic composition of oxygen in their marine samples would reveal a record of ocean temperatures over millennia — in other words, they could measure paleotemperatures using their specimens. For this, they required a reference gas of carbon dioxide (CO2) — prepared from their original PDB sample. Thus, a reference artifact for carbon and oxygen isotopic composition was born.
Eventually, new reference materials were adopted to realize the successor to the PDB isotope delta scale: the internationally agreed-upon Vienna Pee Dee Belemnite (VPDB) delta scale. For a time, those newer materials included the NIST reference material NBS-19 — a limestone ground from a white marble slab in the form of a toilet seat. Over the decades, consistency in these standards has enabled countless studies of isotope ratios here on Earth, as well as studies of the isotopic composition of meteorites and of the Martian atmosphere using an optical instrument onboard the Curiosity rover. But essentially, each of those studies relied upon artifacts made traceable in some way to the original PDB artifact that Lowenstam and Urey dug out of a riverbank during their fossil hunting trip some 70 years ago.
Opportunities in Measurement Science
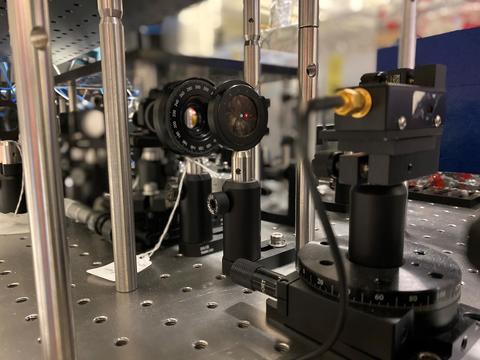
So, can artifact-free measurements of isotopic composition be made traceable to the new SI? In the field of measurement science, traceability is a term used to describe a continuous chain of measurements and methods that together establish a transparent connection between any one measurement and an agreed-upon standard or reference. As a step in that direction, my colleagues and I have published a paper in Nature Physics that reports a value for the stable carbon isotope ratio in VPDB inferred from accurate, calibration-free optical measurements. The optical measurements, led by guest researcher Hongming Yi during his time at NIST, involved the injection of two probing laser beams into a tabletop sample cell of CO2 in air. Using highly reflective, low-loss optics, we were able to create an interaction between our lasers and the carbon dioxide over a distance of many kilometers while keeping the instrument around a meter in size. This increased interaction path length gave us a very precise measurement of the stable carbon isotope ratio in CO2.
Our result agrees well with the values for VPDB derived from other well-calibrated measurement methods, and therefore provides a first independent check on the last several decades of research — dating back to the time of Lowenstam and Urey and their marine sample from that riverbank in South Carolina.
Our work involved collaboration with theoreticians to develop quantum chemistry predictions of the spectroscopic properties of CO2 and was built upon years of prior work to benchmark those predictions against accurate measurements. Abneesh Srivastava, research chemist at NIST, performed state-of-the-art mass spectrometry using an instrument like those found in the most advanced analytical chemistry and forensics laboratories. In addition, our team worked with many other NIST colleagues to use standard reference materials of air with known concentrations of carbon dioxide.
The measurement science community continues to work on the challenge of establishing artifact-free scales. Such scales are applicable to tracking fugitive nuclear emissions caused by nefarious actors, plant decommissioning or industrial accidents. They also have applications in food safety and medicine, including for the real-time tracking of isotopically labeled tracer compounds. Further, the comparison of isotope ratios across both space and time can reveal the origins of our solar system, and potentially the origins of other worlds. For out-of-this-world measurements in cosmochemistry, optical spectroscopy is a convenient choice — with precedent. It was in 1929 that an isotope of carbon was first assigned in an optical emission band of the molecule C2, and subsequent identifications were made in the spectra of distant stars and comets, including spectra of Halley’s comet from its 1910 approach.
For measurements of isotope ratios of the elements, which are important for so many fields, it would be a huge step forward to move beyond calibrating with physical artifacts of limited lifespan that must inevitably be replaced. We aim to devise and disseminate methods that allow for measurements within the framework of the new SI, linking isotopic composition to the fundamental constants of nature. Then, the stability of artifact isotopic composition could be finally tested, improving confidence in isotopic composition scales “for all times, for all people.”